To build complex drop shafts for New Zealand’s Central Interceptor project, RPC Technologies pushed the limits with glass and carbon fiber, vertical filament winding and UV-cure resins. #focusondesign
Multi-material composite vertical shafts. Auckland’s Central Interceptor wastewater and stormwater tunnel is connected to wastewater management, odor treatment and other facilities by a series of vertical shafts. Traditionally made from concrete, eight shafts — up to 7.5 meters in diameter — for this project were designed from fiberglass and carbon fiber composites by RPC Technologies to meet challenging requirements. Photo credit, all images: RPC Technologies Centrifugal Cas Tube
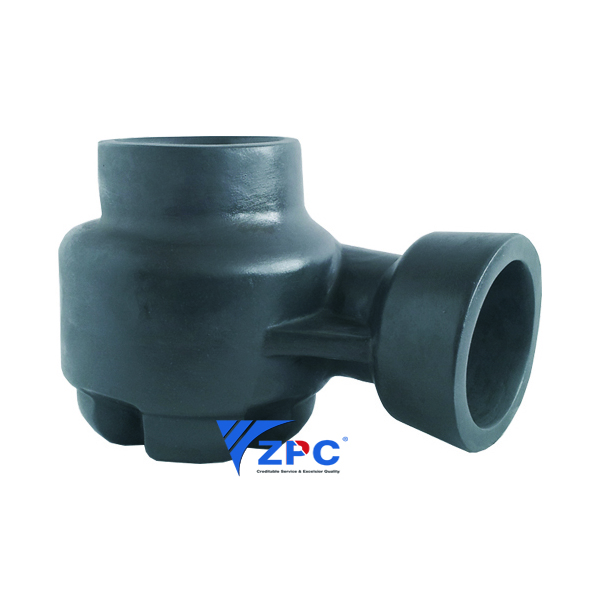
The Central Interceptor (CI) is a massive, 14.7-kilometer, 4.5-meter-diameter tunnel with a 226,000-cubic-meter capacity that, when completed, will run underneath the New Zealand capital of Auckland, collecting waste- and stormwater from smaller connecting pipes around the city and channeling them to the Māngere Wastewater Treatment Plant.
Led by delivery team Ghella Abergeldie JV (GAJV, Auckland, New Zealand) for its customer Watercare (Auckland), this project aims to replace existing aging infrastructure with one central tunnel that is able to handle the growing population of Auckland and alleviate current overflow issues. Begun in 2019, the project is scheduled for completion in 2026. As large as it will be, the CI itself — made from segments of high-density polyethylene (HDPE)-lined concrete — is only one component of the larger system. Servicing the main tunnel are more than 5 kilometers of sewers, a pump station and facilities for odor control, air treatment and wastewater management, all connected by a series of 18 vertical shafts reaching depths of up to 70 meters.
Traditionally, these shafts would be made from concrete, and, in this project, half of the 18 total shafts are. However, several requirements led Watercare to consider alternate materials for at least some of the shafts. For example, the entire project must be designed to last 100 years, including survival of major earthquake events. Moreover, concrete shafts, while generally durable and long-lasting, are subject to corrosion from the sewer chemicals and gases traveling through them, and would need to be repaired and/or replaced multiple times within the needed timeframe.
The CI system. Vertical shafts (yellow) across the city feed wastewater and stormwater into the Central Interceptor (red), ultimately traveling to the Māngere Wastewater Treatment Plant (far right on map).
Corrosion resistance and durability led Watercare to seek composite solutions for some of the vertical shafts that run from the street level down to the CI. For this, Watercare turned to RPC Technologies Pty Ltd. (Seven Hills, New South Wales, Australia), which specializes in design, engineering and manufacturing of glass fiber-reinforced polymer (GFRP) composites, including more than 40 years of experience in developing GFRP water and wastewater management solutions.
Tony Caristo, managing director at RPC, explains that the company was approached to manufacture two of the smaller, 3-meter-diameter shafts in GFRP — the remainder of the shafts would all be constructed from traditional concrete by other companies. RPC pitched several of the larger 4.5- and 5-meter-diameter shafts in composites as well. “It’ll last longer, protecting the tunnel shaft against acid or gases from the sewer. We knew composites were a better long-term solution,” he says. The switch to composites, he adds, saves up to 6 months of construction time for the bigger shafts, removing the need for construction workers to install concrete on site in a confined space, improving worker safety. “We eventually convinced them [GAJV] that it was a good solution, and that it would save them considerable construction time.” In fact, GAJV decided to extend the use of GFRP to even larger shafts.
RPC ended up being contracted to design and manufacture eight vertical shafts. Often identified by the Auckland roads they are installed closest to (see the table below), the shafts range from 3 to 7.5 meters in diameter and depths from 14 to 72 meters. While all of the shafts have the same function and the same base design, differences in diameter, depth, construction methodology and more led to a number of design and manufacturing challenges throughout the project.
At the most basic level, each cascade shaft is designed as a series of vertical tube segments — sometimes called “cans” — from 2.75 to 14.5 meters in length that are bolted together into the final shaft module. Within each segment, a dividing wall, made in two halves adhesively bonded in the middle, separate the “wet” and “dry” sides of the shaft.
Wet and dry. A GFRP wall separates the two halves of each shaft, one filled with cascade shelves (pictured left in above image) to dissipate energy as waste- and stormwater falls through, the other to funnel air to an odor control facility.
Wastewater drops down the wet half of the cascade shaft, ultimately channeling into the CI, while air ventilates through the dry half, to be treated in an odor control facility. In the wet half of the shaft, a series of shelves, called cascade drop structures, are built into the shaft walls. “As the wastewater comes down, it cascades down the shelves, which dissipates the energy as it goes down,” Caristo explains. This helps prevent buildup and overflow and reduces wear on the pipes.
For each shaft, Watercare provided the 3D model design, including dimensions, height and shelf placement, as well as mechanical property requirements. RPC then designed the individual structures and manufactured the components. The design process was extensive and took about a year of back and forth, Caristo says, “because at each stage, the design had to be reviewed with the Watercare engineers.”
To provide a 100-year service life, the shafts needed to meet specific long-term strength, fatigue, abrasion, erosion, external pressure and seismic deformation requirements. The raw materials and part designs were first analyzed via finite element analysis (FEA), followed by a 12-month testing process of test panels and prototypes at RPC’s testing center in Newcastle, outside of Sydney, Australia. This testing process led to several iterations for each of the designs before reaching the final manufacturing steps. An adapted version of test method BS EN 13121-3:2016, developed for GFRP tanks and vessels by BSI Group (U.K.), were used to inform testing.
Shaft components are manufactured between two of RPC’s six total facilities. The smaller shafts — the two 3-meter-diameter shafts and the three 4.5-meter-diameter shafts — are manufactured at RPC’s facility in Batam, Indonesia. The larger, 5.5-meter-diameter and 7.5-meter-diameter shafts are manufactured in Batam and Corio, Victoria, Australia.
For the various segments and shelves, a variety of raw materials are used, Caristo says, including polyester and vinyl ester resins; methyl methacrylate (MMA) and epoxy adhesive; acid-resistant ECR glass fibers as triaxial and biaxial continiuous rovings for the filament-wound tubes; and 0/90 biaxial, ±45 double bias or unidirectional fabrics for the shelves and dividing walls.
The diameter and numbers of segments differs per shaft, but each segment is built from individually designed, filament-wound GFRP (using biaxial or triaxial glass fiber rovings) manufactured on one of RPC’s eight total filament winders supplied by CNC Technics (Hyderabad, India) and McClean Anderson (Schofield, Wis., U.S). Wall thickness varies along each shaft — thinner for segments that will be installed closest to ground level and that see the lightest loads, and thicker the deeper the shaft will be installed underground. For the 7.5-meter-diameter shaft, the deepest segment required a wall thickness of 300 millimeters.
Inside the shafts, the exposed surface of the cascade shelves are lined with HDPE, while the walls and splash zones include silicon carbide (SiC) within a corrosion barrier, to improve wear and abrasion resistance and to further protect the walls against chemicals from the wastewater. A series of GFRP lips are also manufactured directly into the inner walls, onto which the cascade shelves are bonded during assembly.
Corrugated cascade shelves. These shelves are designed as two sandwich panels bonded together to form a corrugated shape to minimize weight.
The cascade shelves, designed to support up to 70,000 kilograms each, are made from two GFRP and foam core sandwich panels made via vacuum-assisted infusion and then bonded together to form a corrugated, hexagonal shape. This shape, developed by RPC in previous wastewater treatment projects, adds torsional stiffness and strength at the lightest weight possible, Caristo says. He adds that to secure the hold, the MMA adhesive is heated in a curing oven to 90°C after application. Each shelf undergoes mechanical testing before assembly into the final shaft.
The tube-shaped segments and cascades are pre-assembled as much as possible at the manufacturing plant, then shipped to the construction site, where segments are bolted together to form the larger modules for installation.
There were a number of design challenges encountered along the way that required analysis and redesign — such as difficult-to-access sewer entry points and very thick laminates for certain modules due to extreme depths — but the biggest challenge, Caristo says, was designing the 7.5-meter-diameter, 69-meter deep, 21-segment-long May Rd shaft. “We’d developed large cascade shafts before, up to 5 meters in diameter or so, but never one this large,” he says. At first, Watercare “didn’t quite believe we could go to such a large diameter with GFRP. But we knew that we had the technical ability to do it.”
Carbon fiber and fiberglass beams. To support the cascade drop shelves over the width of the 7.5-meter-diameter shaft, fiberglass/carbon fiber composite sandwich structures were hand laid and vacuum-infused, then installed in a grid pattern.
One challenge in designing the larger shaft was that the cascade shelves needed a stronger support structure to secure them across the wider diameter. RPC ultimately designed a grid of interlocking beams made from a combination of carbon and glass fiber fabrics infused into composite sandwich panels. RPC typically specializes in GFRP, but in this case, high rigidity and very light weight were needed, and the addition of carbon fiber made the most sense. “This material combination minimizes both weight and cost,” Caristo says.
The main difficulty in the beams’ design, Caristo adds, is that because of the requirement to accommodate potential earthquake activity, “the structure had to be rigid as well as flexible.” The beam grid is designed in two pieces: An “S” shaped joint — which adds flexibility of movement — and “T” shaped beams. These slot together and are laminated to form an integrated structure to be bonded into the shaft module with the cascade shelves installed on top of them.
Another design challenge for the larger shafts was how to filament wind the segments themselves. The parts were too large to be wound on the company’s traditional horizontally oriented filament winder, which has a limit of 6 meters. So, RPC’s Corio, Australia, facility developed an automated, vertical filament winder able to cover the larger size capable of up to 8 meters in diameter. RPC developed the specifications for the system, which was built by partner CNC Technics. This system allowed for manufacture of the taller and wider structure, but also came with several of its own challenges. One was manufacturing ribs on the exterior of the 7.5-meter-diameter shaft. “With a normal horizontal filament winder, the laminate works quite well, it sticks,” Caristo says, “but when you try to do the same thing in a vertical winder, gravity pulls everything down and keeping everything aligned is technically challenging.”
Automated, vertical winding. RPC’s 7.5-meter-diameter May Rd shaft is the largest it has built to date, and wider than the limitations of its typical horizontal filament winders. RPC worked with filament winder supplier CNC Technics to build an automated vertical winder, and used UV-cure resins to enable fast enough cure to prevent resin or fibers from slipping due to gravity.
RPC resolved the issue with the use of an ultraviolet (UV) light curing system and UV-cure polyester or vinyl ester resin (depending on the particular component) “which hardens the resin almost instantly,” he says. This system worked to cure the part before gravity worked on the laminate, and also addressed heat distortion. Winding with traditional resin in such a large, thick laminate can cause heat buildup, Caristo explains, “which potentially could crack some of the laminate.” With this UV-cure method, “you end up avoiding the heat buildup.”
Ultimately, RPC built about half of the 21 total modules for the May Rd shaft in Corio using the vertical winder and UV-cure resins, and half in Batam, Indonesia, using a traditional horizontal winder. Caristo explains that rather than build a second vertical winder, the company decided to adapt the winder in Batam, building a new mandrel to accommodate the larger parts and adapting to UV-cure resin for the ribs to simplify the curing process.
Installing the shafts. The final challenge is installing each shaft module by module underground. RPC aims to have its final shafts installed by August 2024.
The CI project is slated for completion in 2026, but RPC’s shafts are expected to be completely installed by August 2024. “Our structures have to be installed early in the process, because there are a lot of structures that need to be installed on top of these shafts,” Caristo explains. Installation for shafts like these is complex, he adds, with the sheer size of the shafts, depth, visibility underground and site accessibility all playing roles in its success. The overall process is a team effort, requiring close cooperation between RPC, responsible for installation methodology and supervision, and GAJV.
Caristo notes that the Keith Hay Park shaft has been the most challenging so far — at 3 meters in diameter, it is one of the smaller shafts, but also the deepest at 72 meters. “Normally, we start at the bottom of the trench, and install one module at a time, working up,” he says. “But this shaft was so deep, and full of construction site bentonite slurry, that we had to start from the top, and shift down each segment as we went down. It all worked very well, but was a challenge.”
For utility poles and pipes to protective structures and cell towers, composites offer advantages like corrosion resistance, durability and light weight for ease of transport and installation.
QuakeWrap's 16-foot-diameter FRP pipes will transfer more than half of Detroit's sewage from a pump station.
CompoTech’s Compolift technology leverages automated filament winding to provide high-strength, high-stiffness extendable masts for use on mobile surveillance vehicles, boats and more.
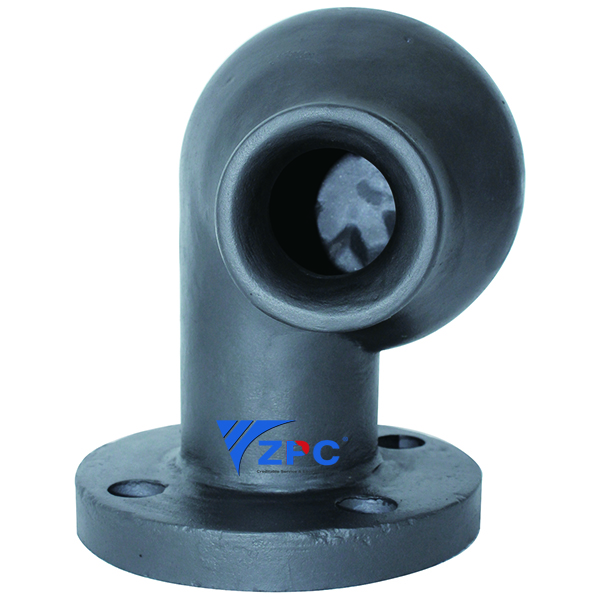
High Temperature Resistance Kiln Furniture © 2023 Gardner Business Media, Inc. Privacy Policy [Log On]